For those working in the chemical industry, achieving operational excellence means more than surface-level improvements. It involves implementing targeted strategies that can significantly enhance plant performance. Let's focus on achieving excellence in the most important key areas.
Scope of Operational Excellence in Chemical Plant
Operational excellence in a chemical plant encompasses the continuous improvement of processes, quality, safety, and efficiency. It aims to maximize productivity while minimizing waste and costs. By integrating advanced technologies, data-driven decision-making, and a skilled workforce, chemical plants can achieve sustainable growth and competitive advantage in a dynamic industry.
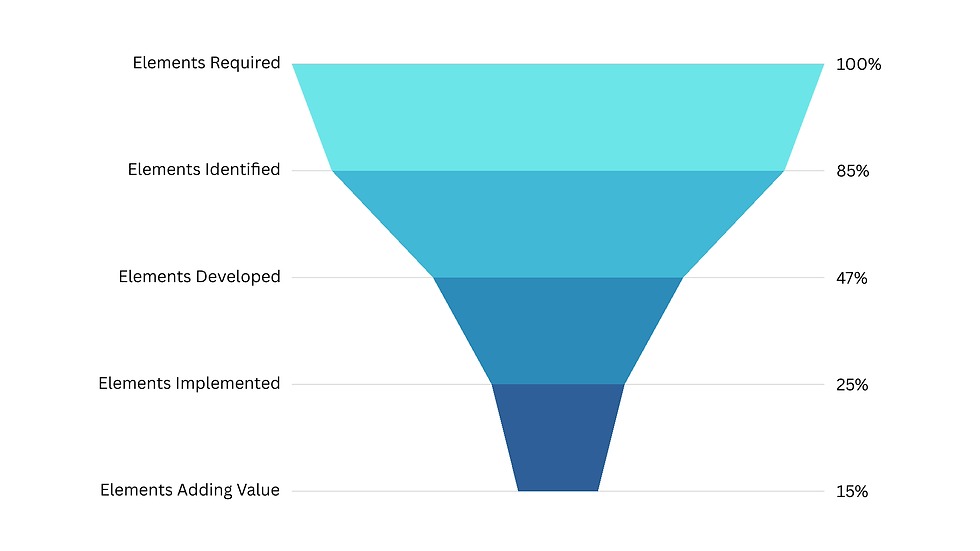
Quality Control: Enhancing Product Integrity with Precision Operational Excellence in Chemical Plant
Quality control in chemical manufacturing is a meticulous process that involves ensuring consistency and reducing variability at every stage of production. Given the complex nature of chemical processes, maintaining tight quality control requires sophisticated tools and a deep understanding of the process.
Achieving operational excellence in chemical plants means proactively going beyond basic quality checks to implement precise, data-driven control systems that align with the overarching business transformation in the chemical industry.
Six Sigma Implementation
DMAIC Framework: Use the DMAIC (Define, Measure, Analyze, Improve, Control) method to systematically decrease process variability. For example, a Six Sigma project in a polymer production line might focus on reducing off-spec products by analyzing data to find critical process parameters (like polymerization temperature) and controlling them more tightly.
Design of Experiments (DoE): Use Design of Experiments (DoE) to optimize key process variables. A practical application could be in a batch reactor, where DoE helps determine the optimal combination of temperature, pressure, and reaction time to maximize yield and minimize by-products.
Gage R&R Studies: Conduct Gage R&R (Repeatability and Reproducibility) studies to ensure that measurement systems are reliable and contribute minimally to process variability. For instance, in a paint manufacturing plant, you could use Gage R&R to check the consistency of viscosity measurements and ensure that the final products meet strict quality standards.
Inline Process Analytical Technology (PAT)
Near-Infrared Spectroscopy (NIR): Integrate NIR spectroscopy for real-time monitoring of critical quality attributes (CQAs) such as moisture content, particle size, or chemical composition. In a pharmaceutical chemical plant, NIR might monitor active pharmaceutical ingredient (API) concentration during synthesis, allowing for immediate adjustments to process parameters.
Raman Spectroscopy: Use Raman spectroscopy for non-invasive, real-time analysis of chemical compositions, particularly in complex mixtures. For instance, in polymer production, Raman spectroscopy can monitor the degree of polymerization, ensuring the final product meets specific molecular weight requirements.
Multivariate Data Analysis (MVDA): MVDA can be applied to analyze complex data sets from PAT systems, enabling the detection of subtle shifts in process conditions that might affect product quality. In a speciality chemicals plant, MVDA can be used to correlate process variables with product quality metrics, driving continuous improvement.
Statistical Process Control (SPC)
Control Charts: Implement control charts (e.g., X-bar, R-charts) to monitor process stability in real time. For instance, in a chloralkali plant, control charts could be used to monitor the concentration of chlorine in the final product, ensuring it remains within tight specifications.
Capability Analysis (Cp, Cpk): Utilize capability analysis to assess process performance relative to specification limits. In a high-purity chemical production facility, Cp and Cpk values can indicate how well a process performs and where improvements are needed, ensuring consistent product quality.
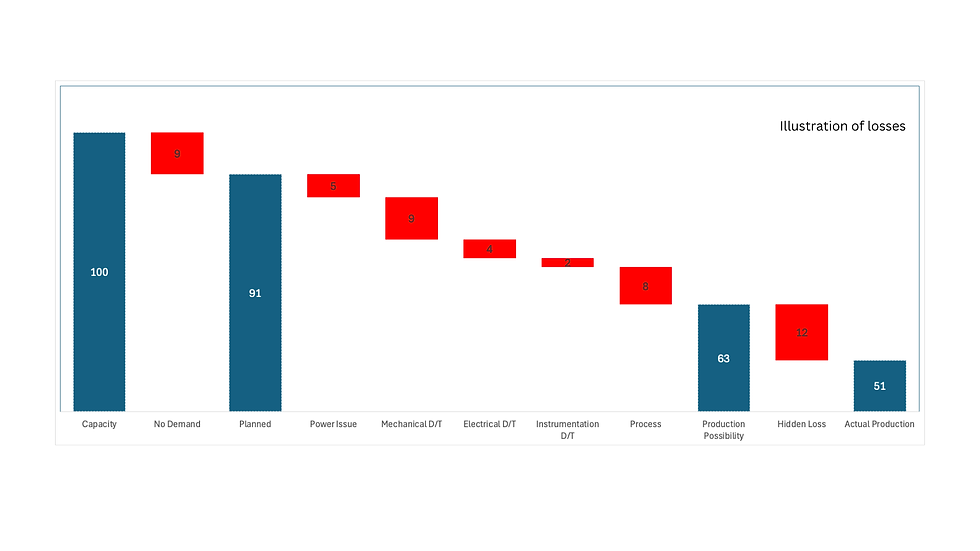
Process Optimisation: Driving Operational Excellence in Chemical Plant
Operational excellence in chemical plants hinges on process optimization, where every unit operation is scrutinized to drive peak performance and inspire potential improvements. This is not merely about tweaking a few variables; it’s about fundamentally rethinking processes to align with business transformation goals in the chemical industry.
Advanced Process Modeling
Simulation Tools: Utilize advanced AI simulation tools to model complex chemical processes, allowing for comprehensive scenario analysis. For example, a petrochemical plant might use simulators to simulate the cracking process, determining optimal temperatures and pressures to maximize ethylene yield while minimizing coking.
Sensitivity Analysis: Conduct in-depth sensitivity analyses to identify which variables (e.g., catalyst concentration, reaction time, or feedstock quality) most significantly impact product yield and purity. Understanding these dependencies allows for targeted process adjustments that enhance overall efficiency.
Real-Time Optimization (RTO): Implement Real-Time Optimization systems that continuously adjust process parameters based on live data. For instance, in ammonia synthesis, RTO can adjust reactor conditions to maintain optimal conversion rates as feedstock quality or environmental conditions change.
Energy Integration
Pinch Analysis: Pinch analysis improves heat exchange systems so that they can use energy as efficiently as possible. This method can find ways to reuse waste heat from chemical reactions to preheat raw materials in a large chemical plant, reducing the need for external heating.
Heat Recovery Systems: Let's update current processes by adding advanced heat recovery systems like Organic Rankine Cycles (ORC) to generate power from low-temperature waste heat. For instance, ORC can be used in sulfuric acid plants to capture and convert waste heat into electricity, which improves the plant’s energy efficiency.
Debottlenecking
Bottleneck Studies: Identify and alleviate bottlenecks through detailed bottleneck studies. These studies focus on throughput limitations caused by equipment capacity, control system constraints, or operator practices. For example, debottlenecking might involve upgrading extrusion equipment to handle higher capacities in a polyethene plant or optimizing catalyst usage to speed up polymerization.
Revamping and Retrofitting: Implement revamping or retrofitting critical equipment, such as upgrading compressors to higher capacity models or installing advanced distillation columns that allow for finer separation with less energy consumption. These changes can increase production capacity without the need for significant capital expenditure.
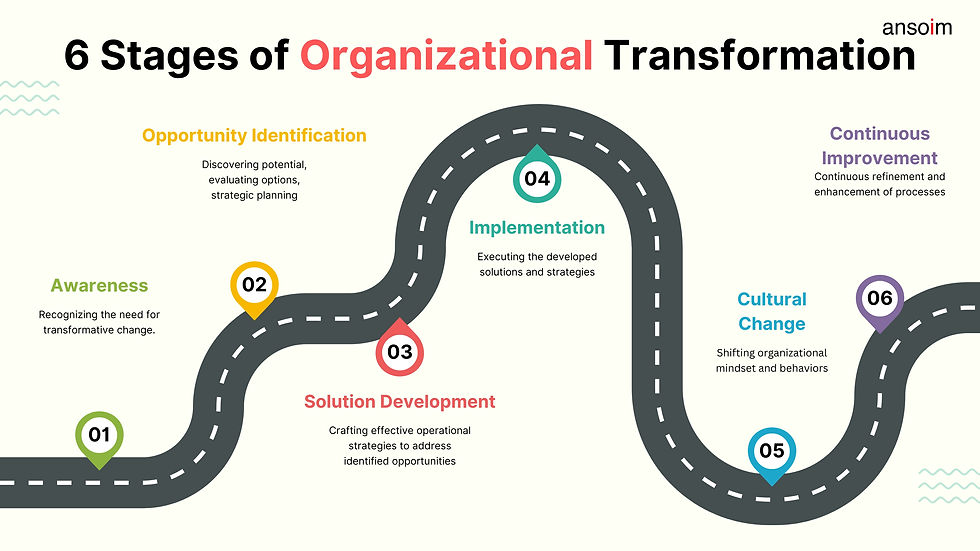
Supply Chain Efficiency: Synchronizing Operations with Demand
Supply chain efficiency in a chemical plant involves an intricate balance of supply, production, and demand.
Supply chain efficiency is a cornerstone of business transformation in the chemical industry. By optimizing logistics and integrating supply chain management with production planning, chemical plants can achieve the operational agility necessary for long-term success.
Integrated Supply Chain Planning
Advanced Planning and Scheduling (APS): Employ APS systems that link production schedules with real-time supply chain constraints. For example, in an agrochemical plant, APS can optimize the production schedule based on seasonal demand fluctuations and raw material availability, minimizing inventory costs and maximizing production efficiency.
Dynamic Safety Stock Models: Create flexible safety stock models that automatically adjust based on changes in demand and the time it takes to receive inventory. For example, the safety stock levels for essential materials like ethylene in a petrochemical plant could be modified based on market predictions and current supply chain conditions. This helps reduce the chance of running out of stock or producing too much inventory.
Vendor-Managed Inventory (VMI)
Onsite VMI Systems: Implement VMI systems where key suppliers manage inventory levels onsite, reducing the burden on plant logistics and ensuring just-in-time availability. For instance, in a large-scale fertilizer plant, VMI could involve suppliers managing stocks of critical raw materials like ammonia, ensuring consistent supply without extensive onsite storage.
Consignment Stock Agreements: Establish consignment stock agreements for critical raw materials, minimizing capital tied up in inventory. This approach can be particularly beneficial in volatile markets where raw material prices fluctuate significantly, allowing the plant to maintain operational flexibility without significant financial exposure.
Logistics Optimization
Route Optimization Algorithms: Use advanced route optimization algorithms to streamline the distribution of finished products, reducing transportation costs and delivery times. For instance, in a speciality chemicals company, route optimization can help coordinate deliveries to multiple customers in different locations, minimizing travel distances and optimizing load efficiency.
Bulk Material Handling Systems: Implement automated bulk material handling systems that transfer materials from storage to process units with minimal manual intervention. This is particularly important in high-volume operations like bulk chemical plants, where efficiently handling large quantities of raw materials can significantly impact productivity and safety.

Maintenance Excellence: Precision and Predictive Operational Excellence in Chemical Plant
Maintenance excellence is critical for sustaining operational excellence in chemical plants. By adopting predictive maintenance strategies, chemical plants can minimize downtime and align their maintenance practices with broader business transformation goals in the chemical industry.
Maintenance in a chemical plant balances reliability with efficiency, ensuring equipment uptime while optimizing maintenance costs. For industry veterans, this involves adopting both predictive and condition-based maintenance strategies.
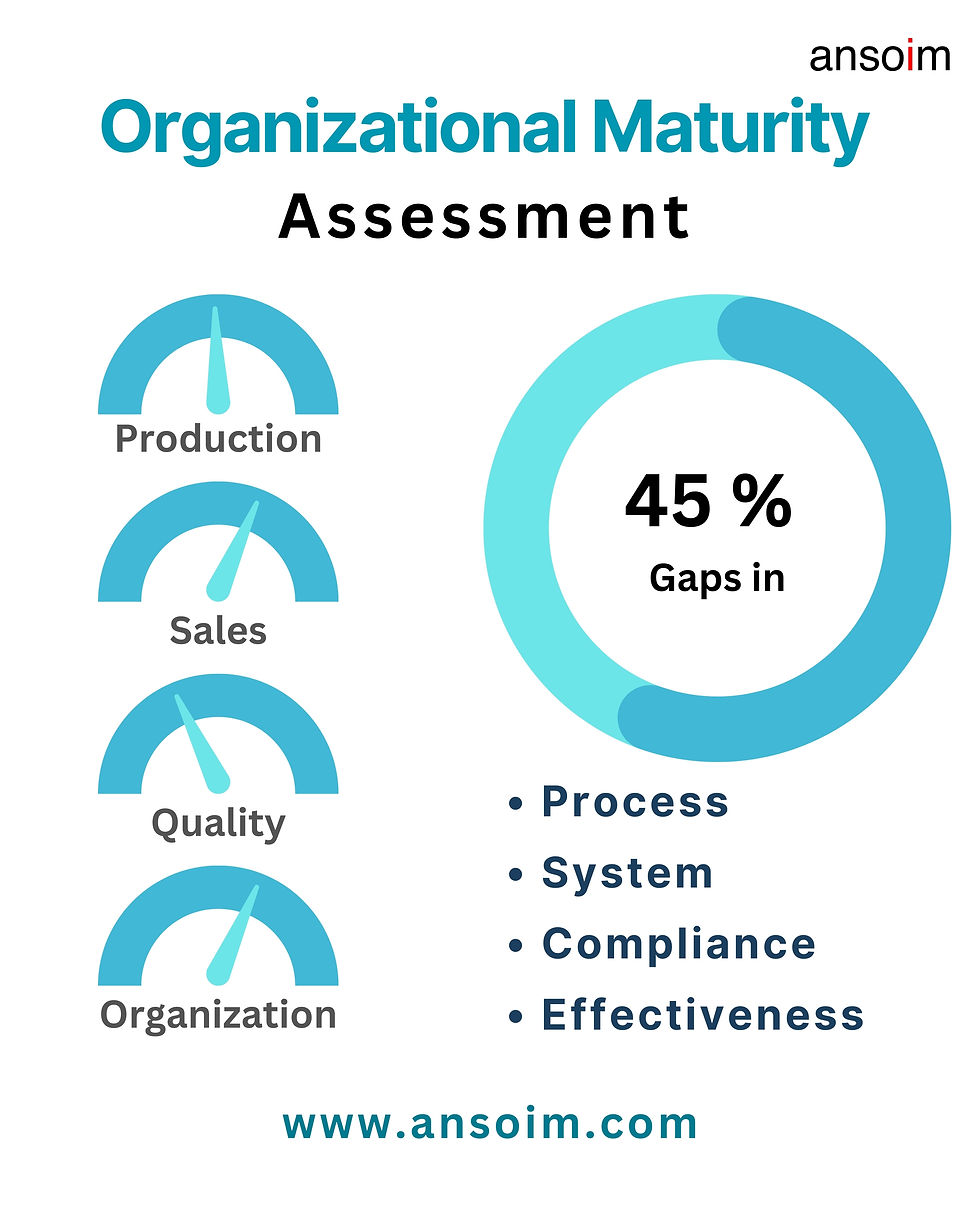
Condition-Based Maintenance (CBM)
Vibration Monitoring: Integrate vibration monitoring into rotating equipment to detect early signs of wear or failure. For example, you can use vibration analysis in a liquid chemical plant to monitor pumps and compressors. This helps identify potential issues like misalignment or bearing wear before they cause serious problems.
Oil Analysis: Implement oil analysis programs to monitor the health of lubricated systems, such as turbines or compressors. Regular oil sampling and analysis can reveal contamination, degradation, or the presence of wear particles, allowing for proactive maintenance actions that prevent equipment failure.
Predictive Maintenance (PdM)
Machine Learning Models: Machine learning is used to study past maintenance data and predict equipment failures before they happen. For example, this could involve analyzing historical vibration and temperature data from critical pumps in a chemical plant. This analysis would help create a model that can forecast potential failures, allowing for timely action to prevent them.
Digital Twin Technology: Digital twin technology creates a virtual model of equipment, which helps predict performance and schedule maintenance. For example, a digital twin of a distillation column can simulate how fouling affects heat transfer efficiency so operators can plan cleaning and maintenance before performance declines significantly.
Criticality-Based Maintenance Strategy
Asset Criticality Ranking (ACR): Develop an ACR system to prioritize maintenance efforts based on the impact of equipment failure on production and safety. For example, criticality ranking in a sulfuric acid plant might identify the main absorber tower as a high-priority asset, directing more frequent inspections and maintenance activities to prevent downtime.
Resource Allocation: Allocate resources to high-priority assets to ensure critical equipment receives attention without over-maintaining less critical items. This approach optimizes maintenance budgets and resources by directing them where they significantly impact plant reliability and performance.

Workforce Training and Development: Building a Competent and Agile Workforce
In the chemical industry, workforce competency is critical for day-to-day operations and for driving continuous improvement and innovation.
A well-trained workforce is crucial for achieving operational excellence in chemical plants. Advanced training programs that align with the strategic goals of business transformation in the chemical industry ensure that employees are equipped to meet current and future challenges.
Competency Management Systems (CMS)
Competency Matrices: Develop competency matrices that map required skills against current workforce capabilities, identifying gaps and training needs. For instance, a competency matrix in a chemical plant that uses advanced control systems might highlight the need for additional training in process control software for certain operators.
Individual Development Plans (IDPs): Create individual development plans (IDPs) for each employee that focus on the skills needed for operational excellence. These plans should align with the plant’s strategic goals to ensure the workforce is ready for future challenges.
Advanced Training Technologies
Virtual Reality (VR): Utilize Virtual Reality (VR) simulations to train operators in complex and hazardous scenarios, enhancing their ability to respond to real-world challenges.
Augmented Reality (AR): Integrate Augmented Reality (AR) into maintenance tasks, providing technicians with real-time guidance and information overlays directly in the field.
Knowledge Management
Centralized knowledge repository: Create a central system for knowledge where employees can easily access lessons learned, best practices, and standard operating procedures.
Cross-functional training: Encourage employees to train across different areas to build a flexible team that can easily switch between roles as needed.
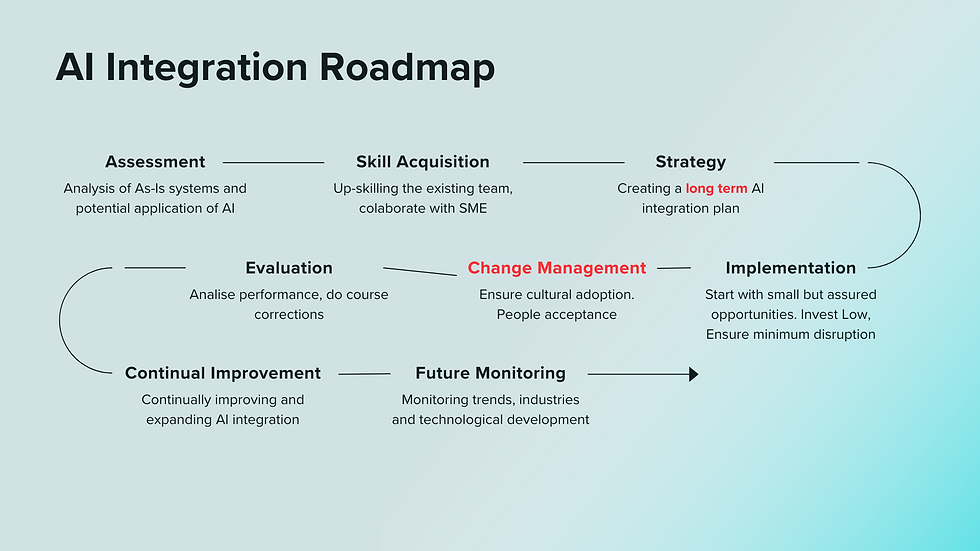
Data-Driven Decision Making: Leveraging Big Data and AI Operational Excellence in Chemical Plant
Data is more than just information in a chemical plant—it’s a strategic asset that drives decision-making and continuous improvement.
Data-driven decision-making is at the heart of operational excellence in chemical plants. By leveraging advanced analytics and AI, chemical plants can make informed decisions supporting day-to-day operations and long-term business transformation in the chemical industry.
Unified Data Platform
Implement a unified data architecture that integrates data from SCADA, MES, ERP, and IoT devices, providing a single source of truth.
Use ETL (Extract, Transform, Load) processes to ensure data consistency and accuracy across all systems.
Advanced Analytics and AI
Deploy machine learning models to predict process outcomes, optimize setpoints, and identify real-time anomalies.
Use predictive analytics for demand forecasting, aligning production schedules with market trends and customer needs.
Process Intelligence
Implement process mining tools to analyze operational workflows, identifying inefficiencies and bottlenecks for targeted improvements.
Utilize digital dashboards that provide real-time visibility into key performance indicators (KPIs), enabling proactive management and rapid response to emerging issues.
Transforming Business Through Operational Excellence
In chemical plants, it’s important to focus on specific areas for driving significant changes. This not only enhances operational performance but also creates a competitive edge in the market.
In the chemical industry, pursuing operational excellence requires continuous learning, adaptation, and innovation. By using your expertise and the advanced strategies mentioned above, you can lead your plant to new heights of efficiency, quality, and profitability.